The focus of our work is creating translatable
polymer solutions for unmet biomedical needs.
Synthesizing functional polymers
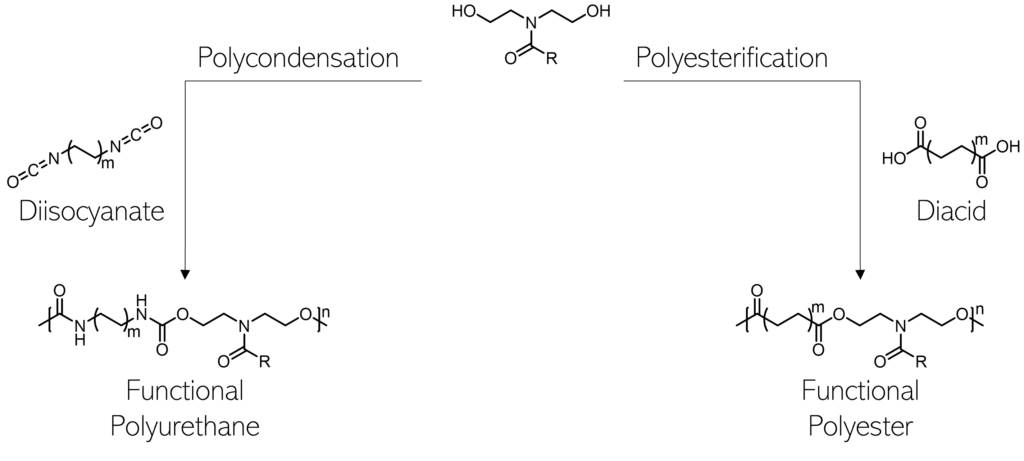
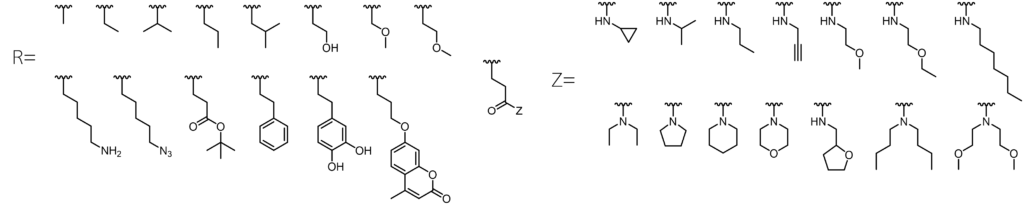
Relevant publications from our lab:
A library of multifunctional polyesters with ‘peptide-like’ pendant functional groups
Peptidomimetic antimicrobial and antibiofilm polymers

According to the World Health Organization, antimicrobial resistance is amongst the top 10 global public health threats. In US alone, data from Centers for Disease Control and Prevention (CDC) claims over 3 million annual antimicrobial resistance infections, that lead to about 50,000 fatalities annually. Since the clinical pipeline for new antimicrobials is running dry, there is a dire need for development of novel antimicrobials. Our group has invested several efforts in designing novel antimicrobial polyesters, polyurethanes, and poly(ester)urethanes that can mimic structural features of host defense antimicrobial peptides, with improved chemical stability and selectivity towards pathogenic bacteria over mammalian cells. We’ve developed antimicrobial polymers for various applications that include antimicrobial surfaces and coatings, decreasing bacterial bioburden in chronic wounds and as adjuvants to common antibiotics to improve therapeutic outcomes. Our ongoing investigation is focused on taking these design principles a step further and modifying the polymer structure to have activity against not just planktonic bacteria, but surface associated bacterial communities called as biofilms, which based on data from US National Institutes of Health (NIH) are responsible for over 65% of all microbial infections.
Overcoming antibiotic resistance
through polymer + drug synergy
Antibiotic-resistant Gram-negative bacteria are emergent pathogens, causing millions of infections worldwide.1,2 The outer membrane (OM) of Gram-negative bacteria plays an important role in protecting it from hydrophobic antibiotics.3 In addition, excessive usage of antibiotics accelerates resistance to these bacteria. There is an urgent need for effective agents to combat antibiotics resistant gram-negative bacteria.
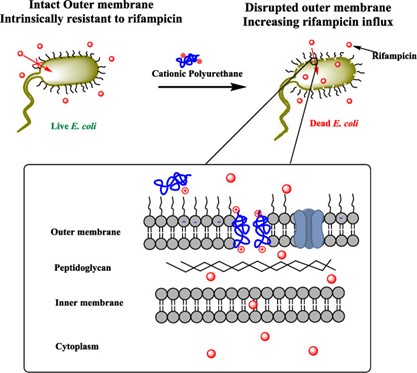
Recent work from our group by Tantisuwanno et al. (doi.org/10.1021/acs.biomac.1c00306) has demonstrated an alternative method to kill E. coli by using rifampicin, an outer membrane excluded antibiotic, in combination with cationic polyurethane. This combination treatment demonstrated a synergistic effect, two agents applied together shows higher potency compared to individual agent applied alone, and could effectively eliminate clinical isolated multidrug-resistant E. coli. Moreover, the composition of these cationic polyurethanes can be tuned to minimize toxicity while maintaining the synergy effect with rifampicin.
Therapeutic delivery via coacervating polymers
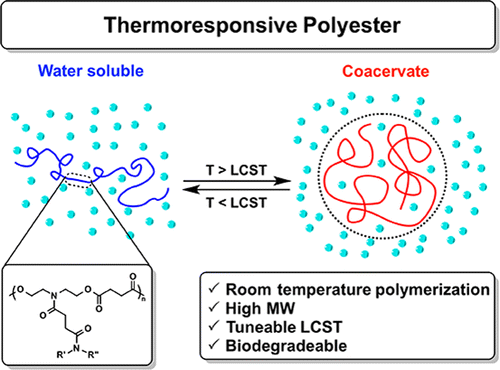
In recent years smart materials have gained popularity as their physical or chemical properties change in response to an external stimulus such as light, heat, pH, or temperature. There is a class of polymers that undergoes a coil-globule transition when the solution temperature is raised above a specific point known as the lower critical solution temperature (LCST). Below the LCST these polymers are soluble, but above the LCST they collapse and dehydrate. If a polymer undergoes an incomplete dehydration, it can collapse into partially hydrated polymer rich nanodroplets with weak intermolecular forces known as coacervates. Over time, the coacervates can coalesce into a polymer rich liquid phase and a polymer poor liquid phase. The polymer righ phase . Our lab has developed a library of materials known as thermoresponsive polyesters (TRPEs) which can form coacervates with varying LCSTs.
There are two common methods for measuring the LCST of a polymer. The most accurate method is by DSC. The presence of an endothermic peak indicates the breaking of hydrogen bonds and the dehydration of a polymer which is entropically favored by the release of water. For coacervating polymers, the incomplete dehydration results in the lack of an endothermic peak in a DSC curve, so the second method is used to approximate the LCST. Below the LCST, the solution of polymer is clear. As the solution is brought above the LCST, the solution becomes turbid due to the formation of coacervate nanodroplets. By measuring the turbidity and transmittance of light through the solution, the cloud point temperature (Tcp) can be determined. The Tcp is defined as the point where the solution reaches 50% transmittance.
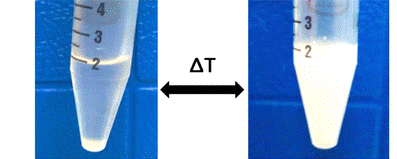
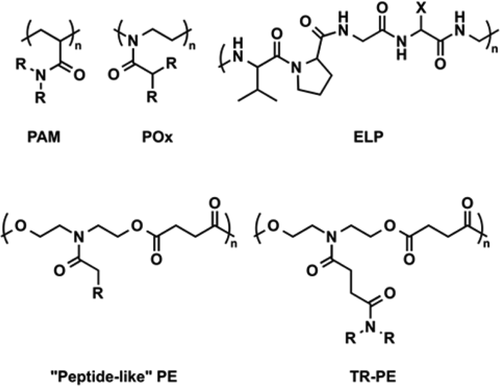
Coacervates are particularly attractive for biological use as the partial dehydration of the polymer results in minimal conformational changes and prevents denaturation which makes them useful for encapsulating both hydrophobic and hydrophilic targets. The minimal change can be utilized to protect proteins or active drugs whose functions rely on their structure. Past literature has reported other polymers such as elastin-like peptides (ELPs), and polyoxazolines (POxs) as coacervating polymers for target encapsulation. However, biodegradability is critical for polymer use in biomedical applications and ELPs and POxs do not readily degrade under physiological conditions. Our TRPEs utilize the polyester backbone to be readily hydrolysable at body temperature in water.
We have developed libraries of monomers whose homopolymers have Tcps between 0℃ and 100℃. Using this information we can design our polymers to have specific LCSTs making them usable for a variety of applications. We have demonstrated the encapsulation of the cancer drug doxorubicin, and fluorescently labeled bovine serum albumin (FITC-BSA) within our coacervating TRPEs. We have also developed a coacervating underwater adhesive inspired by the coacervating adhesive proteins of sandcastle worms and mussels. By utilizing differences in the Tcp of two polymers we can even make a multilayered coacervate.
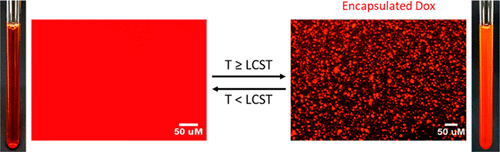
Our lab is currently investigating the molecular interactions between the coacervates and the molecules we want to encapsulate. Using various nuclear magnetic resonance (NMR) techniques, circular dichroism (CD), fluorescence microscopy, rheology, and infrared spectroscopy (IR) we want to learn more about how our polymers interact with encapsulation targets in the coacervate phase.
Relevant publications from our lab:
Efficient Protein Encapsulation within Thermoresponsive Coacervate-Forming Biodegradable Polyesters
The effect of pendant group structure on the thermoresponsive properties of N-substituted polyesters
A Coacervate-forming Biodegradable Polyester with Elevated LCST based on Bis-(2-methoxyethyl)amine
A library of thermoresponsive, coacervate-forming biodegradable polyesters
A library of multifunctional polyesters with ‘peptide-like’ pendant functional groups
3D printed polymer scaffolds for wound healing
Three-dimensional (3D) printing has been widely used in tissue engineering due to its capability in fabricating complex bioengineered tissue constructs, such as ears, heart valves, and bones. Extrusion-based direct-write 3D printing (EDP) is one method that enables printing low modulus materials with porous structure and without the addition of solvent, which allows us to further extend its application in wound dressing.
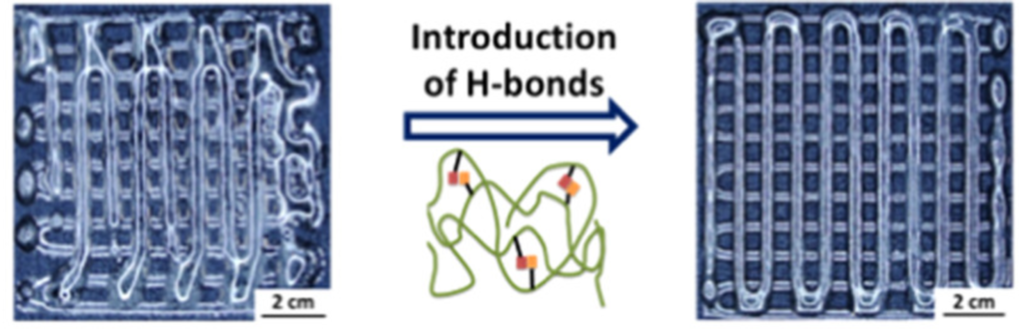
Shape fidelity and printability are two key points to achieve desired constructs without compromising their complexity. Recent works led by Qianhui Liu have demonstrated how H-bonding affects the properties of materials and further maintains the shape fidelity of 3D printed scaffolds. By an in-depth study of rheology (Macromolecules 2018, 51, 9294−9305), we found that side-chain flexibility and hydrogen bonding interactions can be used as a strategy to design materials with desired rheological properties. In another work (Macromolecules 2020, 53, 3690−3699), with increased amounts of H-bonding on the side chains, extruded filament deformation was impeded, leading to enhanced shape fidelity.
Fundamental studies on rheological behaviors on N-substituted polyesters are also established. Printability and rheology were thoroughly examined by varying the functional groups on the side chains. By increasing the side chain length (Polym. Chem., 2019, 10, 5543), the longer chain lengths serve as internal diluents, which allow materials to be extruded at a lower temperature. On the other hand, a library of polyesters was investigated (ACS Appl. Polym. Mater. 2021, 3, 6618–6631). The rheological characterization suggests that these N-substituted polyesters are unentangled melts that allow materials to be printed at ambient temperature without any additive or solvents.
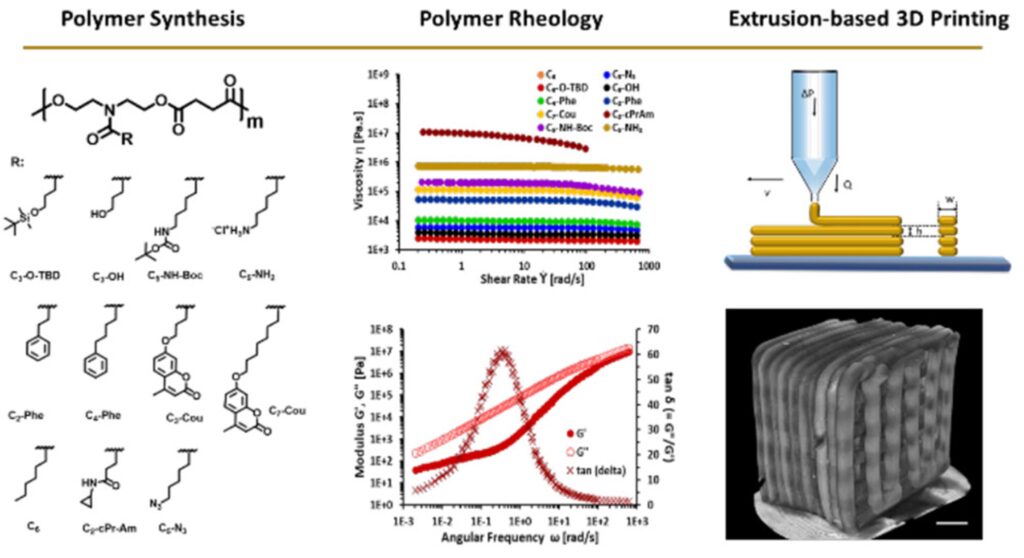
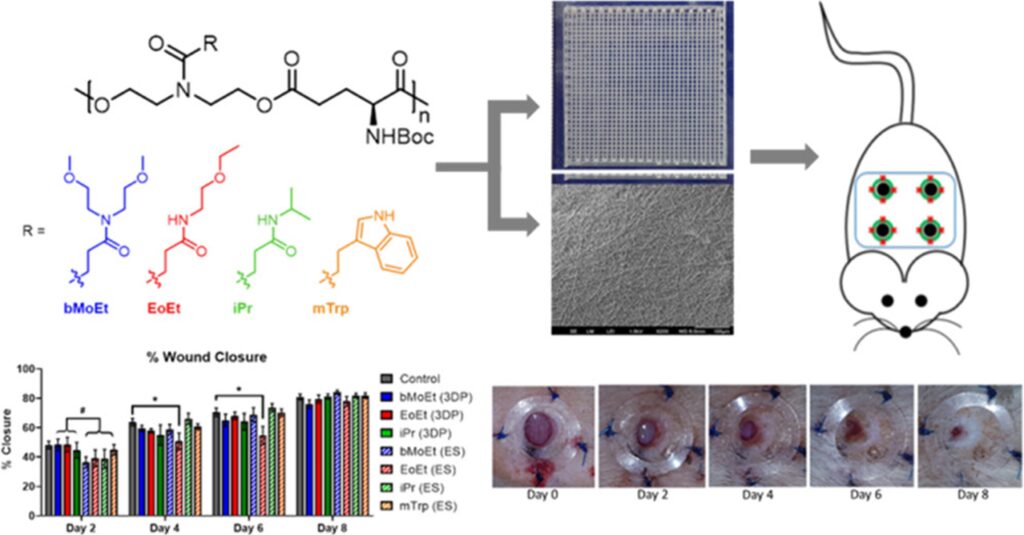
Wound dressing with different thread sizes and polymer compositions are vital factors for wound healing. Polymers with four different side chains are being fabricated into wound dressings with either 3D printed scaffolds or electrospun mats (Biomacromolecules 2020, 21, 10, 4030–4042). The polymer compositions slightly affect the outcomes, such as epidermal thickness and granulation tissue. Moreover, enhanced angiogenesis was seen in 3D printed dressings compared to those treated with electrospun dressings.
Related publications from our lab:
Role of pendant side-chain length in determining 3D printability
Polyester based tissue adhesives
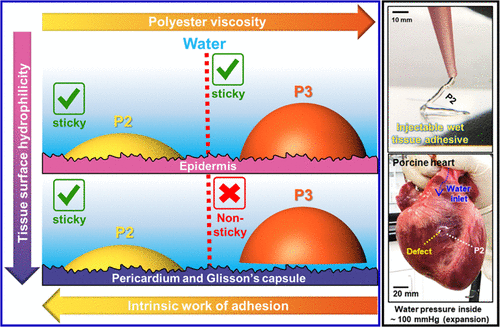
Adhesion of an adhesive to a substrate involves the maximizing both the adhesive interactions between the substrate and adhesive and the cohesive interactions in the bulk of the adhesive material. Therefore, an adhesive has to address the opposing forces of forming good contact with the substrate and having bulk mechanical strength. In addition, in a biological environment, addition of any organic solvent to the adhesive to increase its contact with the substrate is not an ideal option. Tissue adhesives that are too hydrophilic will not make good contact with the substrate and those that make contact due to, or after a chemical reaction, are likely to cause tissue inflammation or damage. In the Joy Lab we have developed tissue adhesives that have remarkable adhesion in wet environments due to their ability to maximize both adhesive and cohesive interactions and by their ability to displace the water layers that are intimately associated with a hydrophilic surface. Our studies have shown that these polyester adhesives have better performance than commercial protein-based adhesives and are degradable and non-toxic. These adhesives have applications are tissue adhesives for wound dressings, skin grafts, dental applications and in engineering applications for underwater adhesives.
Related publications from our lab:
Single Chain Hydration and Dynamics of Mussel-Inspired Soybean-Based Adhesive
Light-Activated Adhesion and Debonding of Underwater Pressure-Sensitive Adhesives
Design principles for creating synthetic underwater adhesives
Advances in Photoreactive Tissue Adhesives Derived from Natural Polymers
Viscosity Attunes the Adhesion of Bioinspired Low Modulus Polyesters to Wet Tissues